Unpublished Opinions
Clinton is an accredited writer for numerous publications in Canada and a panelist for talk radio across Canada and the United States
Gravity Manipulation? Perhaps Breakthroughs Hide in the Fringes?
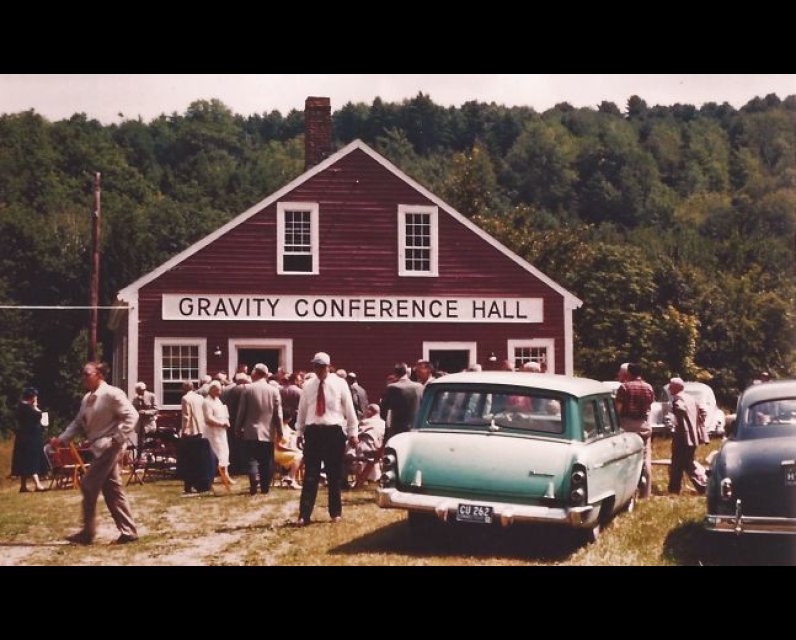
Could theories like string theory have been deliberately pushed to divert attention for defense or security reasons related to gravity manipulation? Is it possible that mainstream physics and chemistry have been steered off course?
People around the globe are increasingly curious about whether UFOs or UAPs from another planet have visited Earth. Over the past five years, leading academic institutions and reputable news outlets on every continent have covered this topic extensively. In life, the simplest explanation is often the most plausible one which often leads to the idea it’s just a misunderstanding of events and odd timing. But what if the academic fields of physics and chemistry have been subtly misled over recent decades? On January 18th to January 23rd 1957, some of the world’s leading physicists & chemists gathered in Chapel Hill, North Carolina, in the United States for something called the “Gravity Conference.” Funded partly by the U.S. Air Force via the Institute for Field Physics. It was an era where the cold-war was at its peak - perhaps breakthroughs hide in the fringes?
Maybe the so-called reports of UFO’s and UAP’s supposedly zipping around aren’t aliens but someone’s secret gravity tech? By the mid 1960’s academic science discussions around gravity sort of went dark.
Could theories like string theory have been deliberately pushed to divert attention for defense or security reasons related to gravity manipulation? Is it possible that mainstream physics and chemistry have been steered off course? Or that groundbreaking new discoveries in these fields have been developed in secret, kept hidden from the academic world? I don’t have the answers. After months of wrestling with these questions, I decided to design two thought experiments to explore whether such possibilities might hold any truth.
These ambitious and intriguing gravity manipulation experiments blend cutting-edge fields like superconductivity, plasma physics, and gravitational research. The theoretical foundation draws on speculative gravito-electromagnetic ideas, aiming to detect gravitational anomalies that could arise from interactions between oscillating superconductors, terahertz radiation, and rotating plasma.
Superconductor Choices (BSCCO and YBCO): These high-T_c superconductors (especially BSCCO) are well-chosen for their unique properties at cryogenic temperatures. The combination of BSCCO's high-T_c and YBCO’s high current density is ideal for probing potential quantum effects in their interactions with electromagnetic fields. I believe oscillating superconductors should be tested as it might interact better with gravity waves.
The idea of using a terahertz source to excite the superconductors and induce oscillations in their plasma modes is well-founded. The THz frequencies are known to resonate with Josephson plasmons, and this could, in theory, amplify nonlinear interactions. The amplitude (100 kV/cm) seems appropriate for initiating measurable effects without overwhelming the system.
Rotating Plasma Lattice is my own interesting thought twist. By embedding superconductors into a rotating plasma structure, the experiment introduces a dynamic electromagnetic environment that could potentially enhance any subtle effects. The rotation adds an intriguing variable that could be important, and the torque from the spinning structure might influence the superconducting system in interesting ways.
The use of high-sensitivity gravimeters (<1 μGal resolution) to measure any gravitational anomalies would be a smart choice to consider, as it provides a sensitive and direct method to test for tiny deviations that could be linked to gravitational shifts.
These experiments if successful would blend cutting-edge superconductivity research with exploratory physics, offering a framework to probe gravity-superconductor interactions, though success is not guaranteed given how little we know about the topic.
If an oscillating superconductor for a Bismuth strontium calcium copper oxide (BSCCO) lattice or Yttrium barium copper oxide (YBCO) lattice combined with rotating plasma and high levels of alternating current tweaks space-time even slightly, it’d be a groundbreaking hint at gravity manipulation. It would permanently change the world as we know it; changing the world of commerce overnight in the realms of transportation and fossil fuels. So any university-level physics and chemistry students with access to an advanced laboratory should livestream these experiments and let all of us know if anything of note happens. It could all be a giant waste of time, or you could be remembered as the new Albert Einstein and Nikola Tesla
Experiment 1: Gravity Manipulation with Oscillating BSCCO Superconductor
Objective
To investigate potential gravitational anomalies induced by a terahertz-driven oscillating BSCCO superconductor interacting with a rotating plasma lattice structure.
Materials and Equipment
- BSCCO Thin Film: 100 nm thick, high-purity Bi₂Sr₂CaCu₂O₈₊δ deposited on a glass substrate (chosen for its high critical temperature and layered structure).
- Liquid Helium Cryostat: Maintains 4 K to achieve superconductivity (BSCCO’s Tc is ~110 K, but lower temperatures enhance coherence).
- Terahertz Source: Free-electron laser or quantum cascade laser (0.5–1 THz, field strength up to 100 kV/cm) for driving oscillations.
- Plasma Generator: Argon gas plasma source with RF coil (13.56 MHz) to create a rotating plasma sheath.
- Rotating Lattice Structure: Copper ring (10 cm diameter) with embedded BSCCO pellets, mounted on a high-precision motorized spindle (up to 10,000 RPM).
- Gravimeter: High-sensitivity gravimeter (resolution < 1 μGal) to detect local gravity changes.
- Magnetic Field Coils: Helmholtz coils generating a uniform 0.1 T field to stabilize the superconductor.
- Vacuum Chamber: Operating pressure < 10⁻⁶ torr to minimize air interference.
- Data Acquisition System: High-speed oscilloscope and sensors for monitoring THz, plasma, and gravimetric data.
Step-by-Step Setup and Procedure
- Prepare the BSCCO Sample:
- Deposit a 100 nm BSCCO thin film on a 5 cm x 5 cm glass substrate using pulsed laser deposition for uniformity.
- Embed BSCCO pellets (1 cm³ each) into the copper ring to form a lattice structure, ensuring electrical continuity via silver paste contacts.
- Set Up the Cryostat:
- Place the BSCCO thin film and lattice ring inside the liquid helium cryostat.
- Cool the system to 4 K over 2 hours, monitoring temperature with a thermocouple to confirm superconductivity via resistance drop.
- Configure the Terahertz Source:
- Position the terahertz source 10 cm above the BSCCO film, aligned perpendicularly to induce oscillations in the Cooper pair density.
- Set the frequency to 0.75 THz (mid-range for resonance with BSCCO’s plasma frequency) and amplitude to 100 kV/cm.
- Establish the Rotating Plasma:
- Introduce argon gas into the vacuum chamber at 10⁻³ torr.
- Activate the RF plasma generator to create a plasma sheath around the rotating lattice ring.
- Spin the ring at 5,000 RPM initially, using the motorized spindle to induce a toroidal plasma flow.
- Apply Magnetic Stabilization:
- Energize the Helmholtz coils to produce a 0.1 T field parallel to the BSCCO film, minimizing vortex pinning disruptions.
- Conduct the Experiment:
- Turn on the terahertz source to oscillate the BSCCO superconductor, driving nonlinear Josephson plasma waves.
- Increase the lattice ring’s rotation to 10,000 RPM over 10 minutes, observing plasma dynamics via optical spectroscopy.
- Position the gravimeter 20 cm above the setup to monitor gravitational acceleration every 1 second for 30 minutes.
- Record baseline gravity, then compare with readings during full operation.
- Data Collection and Analysis:
- Log terahertz field strength, plasma density (via Langmuir probe), rotation speed, and gravimetric data.
- Look for deviations in gravitational acceleration (>1 μGal) correlated with terahertz and plasma activity.
- Repeat the experiment 5 times, varying THz frequency (0.5–1 THz) to identify resonance effects.
The terahertz-driven oscillations in BSCCO may couple with the rotating plasma’s electromagnetic field, potentially altering local spacetime curvature via speculative gravito-electromagnetic interactions.
- Indicators: Small reductions or anomalies in gravitational force (e.g., 0.001–0.1% change), as seen in some unverified superconductor-gravity studies.
- Challenges: Distinguishing signal from noise (thermal, mechanical, or electromagnetic) would require careful calibration.
Experiment 2: Gravity Manipulation with Oscillating YBCO Superconductor
Materials and Equipment
- YBCO Thin Film: 200 nm thick YBa₂Cu₃O₇₋ₓ on an MgO substrate (Tc ~93 K, high critical current density).
- Liquid Nitrogen Cryostat: Maintains 77 K for superconductivity.
- Terahertz Source: Same as Experiment 1 (0.5–1 THz, 100 kV/cm).
- Plasma Generator: Helium gas plasma source with RF coil (13.56 MHz) for a lighter, more responsive plasma.
- Rotating Lattice Structure: Aluminum ring (15 cm diameter) with YBCO pellets, mounted on a spindle (up to 12,000 RPM).
- Gravimeter: Same as Experiment 1.
- Magnetic Field Coils: 0.05 T field for vortex lattice control.
- Vacuum Chamber: Same as Experiment 1.
- Data Acquisition System: Same as Experiment 1.
Step-by-Step Setup and Procedure
- Prepare the YBCO Sample:
- Fabricate a 200 nm YBCO film on a 6 cm x 6 cm MgO substrate using magnetron sputtering for high crystallinity.
- Attach YBCO pellets to the aluminum ring, forming a lattice with conductive epoxy.
- Set Up the Cryostat:
- Place the YBCO film and lattice ring in the liquid nitrogen cryostat.
- Cool to 77 K over 1 hour, verifying superconductivity with a four-point resistance probe.
- Configure the Terahertz Source:
- Align the terahertz source 15 cm above the YBCO film, set to 0.5 THz initially (near YBCO’s Josephson plasma resonance).
- Adjust amplitude to 100 kV/cm.
- Establish the Rotating Plasma:
- Fill the vacuum chamber with helium at 10⁻³ torr.
- Activate the RF plasma generator to form a plasma sheath encircling the rotating lattice.
- Start the spindle at 6,000 RPM, increasing to 12,000 RPM over 15 minutes.
- Apply Magnetic Stabilization:
- Use Helmholtz coils to apply a 0.05 T field perpendicular to the YBCO film, optimizing vortex lattice stability.
- Conduct the Experiment:
- Activate the terahertz source to induce oscillations in the YBCO superconductor, amplifying plasma waves.
- Ramp up rotation speed while monitoring plasma rotation via Doppler shift measurements.
- Position the gravimeter 25 cm above, collecting data every 0.5 seconds for 45 minutes.
- Establish a baseline, then compare with active conditions.
- Data Collection and Analysis:
- Record THz parameters, plasma characteristics, rotation speed, and gravimetric readings.
- Analyze for gravitational anomalies (>1 μGal) linked to oscillation and plasma dynamics.
- Perform 5 runs, adjusting THz frequency (0.5–1 THz) and rotation direction (clockwise/counterclockwise).
YBCO with a higher critical current and oxygen-dependent lattice may enhance gravito-electromagnetic effects when oscillated and coupled with plasma rotation.
- Indicators: Subtle gravity shifts or anomalies, potentially larger than BSCCO due to YBCO’s robustness.
- Challenges: YBCO’s sensitivity to humidity and defects may introduce variability; plasma stability at higher speeds is critical.
Theoretical Basis and Caveats
- Superconductor Dynamics: Both BSCCO and YBCO exhibit Josephson plasma waves under THz excitation, potentially amplifying nonlinear effects. Research on cuprate superconductors suggests these oscillations could interact with external fields in unexpected ways.
- Plasma and Rotation: Rotating plasma may generate a magnetic moment or ionic currents, hypothesized to couple with spacetime per gravito-Maxwell theories (e.g., weak-field approximations of general relativity).
- Gravity Modification: Claims of gravity shielding (e.g., Podkletnov’s experiments) remain unverified. These setups test similar principles but prioritize measurable, repeatable data over speculative leaps.
- Limitations: No established theory predicts significant gravity manipulation at lab scales. Results may reflect instrumental artifacts or subtle electromagnetic interference rather than gravitational effects.
Safety and Practical Notes
- Cryogenic Handling: Use insulated gloves and venting systems for liquid helium/nitrogen.
- Terahertz Safety: Shield personnel from high-intensity THz radiation.
- Plasma Containment: Ensure vacuum integrity to prevent arcing or gas leaks.
- Calibration: Pre-test all sensors in isolation to establish noise floors.
Comments
Be the first to comment